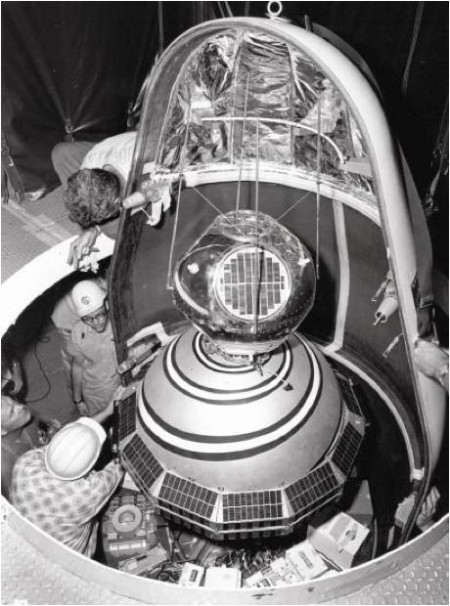
Fifty-two years ago this month, the Transit 2A and GRAB 1 satellites were successfully launched into orbit by a Thor Able Star launch vehicle. This marked the first time that multiple functional satellites were orbited on the same mission.
On Wednesday, 22 June 1960, Thor Able Star 281 roared away from LC-17B at Cape Canaveral, Florida with the Transit 2A and GRAB 1 satellites on board. Both orbiters were designed and developed under the auspices of the United States Navy. Each satellite was successfully placed into its respective target orbit by the powerful hybrid Thor rocket system.
Transit was the first operational satellite navigation system. More formally known as the Navy NAVSAT (Navigation Satellite) System, Transit provided accurate global position data in support of naval worldwide sea operations. The navigation of submarines and surface ships was greatly aided by Transit-provided data as were sundry hydrographic and geodetic surveying programs.
Transit was developed for the Navy by the Applied Physics Laboratory of the Johns Hopkins University (JHU/APL). Work began in 1958 and launch of the first prototype Transit satellite, Transit 1A, took place in September 1959. A number of Transit satellite launches took place over the next 5 years with the system going operational in 1964.
Transit satellites provided position data that was accurate to within about 3 feet. The system revolutionized global navigation and was ultimately used by an untold number of ships and boats. Transit navigational operations ceased in 1996 with the advent of the Global Positioning System (GPS). One of the great benefits of GPS is that position data are provided continuously whereas Transit provided discrete data about once an hour.
The other notable passenger aboard Thor Able Star 281 was the GRAB 1 satellite. GRAB (Galactic Radiation Background Experiment – go figure) holds the distinction of being the first successful Electronic Intelligence(ELINT) satellite in history. Stationed in a 500-mile orbit, its mission was to monitor Soviet radar emissions for the purpose of mapping that country’s air defense radar system. GRAB also monitored electronic activity coming out of Red China and other communist nations as well.
GRAB transmitted reconnaissance information obtained during a data collection pass to special ground stations within its field of view. These data were recorded and found their way to military and civil security agencies for analysis. For obvious reasons, the GRAB program was classified. In fact, it was not until 1998 that the program was declassified.
A confusing aspect of the GRAB 1 satellite is that it is often referred to as the SOLRAD 1 satellite. (SOLRAD stood for Solar Radiation.) This confusion was in fact intentional. The GRAB/SOLRAD satellite in actuality had two scientific packages on board. The publicly-known entity was SOLRAD. As the name implies, its purpose was to gather solar radiation data. Its alter purpose was as a front for the clandestine ELINT mission of the GRAB package.
The dual mission of the GRAB/SOLRAD 1 satellite was very successful. GRAB ELINT operations took place over a 3 month period and included a total of 22 data collection passes over denied territory. In its 10-month operational mission, SOLRAD gathered an extensive amount of solar emissions data which, when correlated with ground measurements, added significantly to our understanding of the Sun.
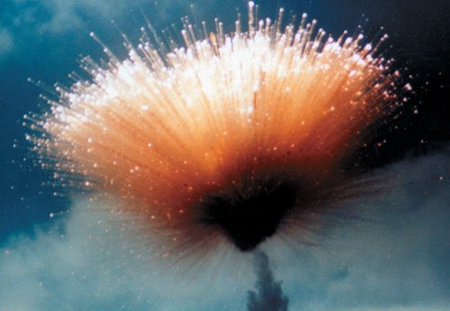
I recently came across this thought-provoking excerpt while conducting final research for our soon to be released Aerospace Lessons-Learned course. These are sobering words, yet extremely accurate. As aerospace professionals, striving for advances in a field fraught with the unknown, we will encounter failure. Without a doubt, we will fail. However, we can minimize the frequency of those failures and thus maximize our rate of success by learning from the past.
You will fail. It is important to understand this certainty. As an aerospace engineer, you will be building vehicles that fly under conditions never before encountered. You will, despite all the analysis and efforts put into the projects, still be voyaging into the unknown. You do not know what you will find. In ancient times, much of the world was a blank. Mapmakers would mark these unknown places with the words “Here There Be Dragons.” Despite all the advances in the first century of heavier-than-air flight, you need to understand that the dragons are still out there. You will fail.
That you will fail is certain. The causes of the failure you will experience, however, may be entirely unknown, and will range from the overarching to the mundane. A failure may come because, with existing knowledge and technology, the goal was unattainable. It may occur due to changing social factors, national needs, and aerospace policies. The failure may come as a result of a grave mismatch between budget and tasks at hand, or a lack of necessary political commitment. The failure may occur because an assumption was made that should have been challenged, because a modification was made that was better left undone or a question went unasked, or some flaw went undetected. And, perhaps worst of all, the failure may occur for reasons you will never truly understand.
While you are being trained in the skills needed to successfully build an aerospace vehicle, you may be less well prepared for events that can occur in the wake of a failure. On the personal level, you will have to deal with the emotional impact of having spent years working on a project, having missed evenings, weekends, and holidays with family and friends, of having overcome an endless series of problems and setbacks, only to see it all fail within a matter of seemingly random and dispassionate seconds.
This wrenching but instructive experience will then be followed by a mishap investigation, which may continue for months. During this time, the project is no longer under the control of project personnel. The investigators are the ones running things. You and the other project personnel have little input into what is done in the course of the effort. You may also face uncertainty over the project’s future in the wake of the failure. As with the immediate aftermath, this will take a toll on you and other project personnel.
The question is not if you will fail. Instead, the question is how you will deal with that failure, and how you will overcome it.
Excerpt above is from Road to Mach 10: Lessons Learned from the X-43a Flight Research Program written by Curtis Peebles, aerospace historian for the Smithsonian Institute.
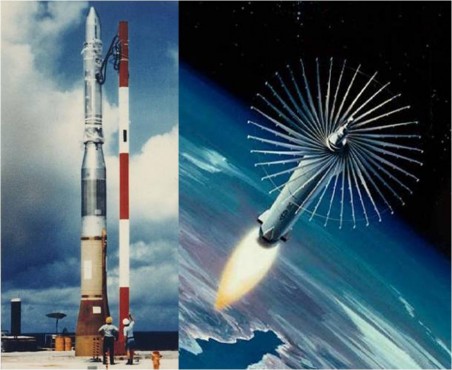
Twenty-six years ago this month, the United States Army’s Homing Overlay Experiment (HOE) anti-missile successfully intercepted a ballistic missile target in space. The feat marked the first time in aerospace history that an exoatmospheric hit-to-kill intercept was achieved.
The HOE Program was a technology demonstration effort conducted by the United States Army (USA) to enable a nonnuclear hit-to-kill intercept capability for application against Soviet nuclear warheads. The Lockheed Company was awarded the development contract in 1978. A total of four (4) exoatmospheric flights tests were conducted from February 1983 through June 1984.
The HOE interceptor was unique in that it employed a 13-ft-diameter radial net that markedly increased the frontal area of the interceptor. The net mechanism was deployed just before target intercept. This unit consisted of 36 aluminum spokes, to each of which was affixed a trio of stainless steel weights or fragments.
The HOE test vehicle was equipped with an IR seeker for target detection in space. Upon target detection, the onboard propulsion system was driven by vehicle guidance and control to place the interceptor on a collision course with the target. At a closing velocity of 20,000 ft/sec, the kinetic energy of the 2,600-lb HOE interceptor was more than sufficient to destroy the target.
A two-stage Minuteman 1 Intercontinental Ballistic Missile (ICBM) served as the HOE booster. It was launched from Meck Island on the Kwajalein Missile Range out in the Marshall Islands. The target vehicle was also a Minuteman missile configured with a dummy warhead. It was launched from 4,500 miles away at Vandenberg Air Force Base, California.
The first three (3) HOE flight tests failed to produce a successful hit-to-kill intercept due to system detection and guidance anomalies. However, on Sunday, 10 June 1984, everything worked as planned when the fourth and final HOE test vehicle successfully intercepted and destroyed the ballistic target via kinetic kill. In the glow of the post-flight celebration, the successful HOE intercept was likened unto hitting a bullet with a bullet.
The HOE flight demonstration success came at a pivotal time in that the vaunted Strategic Defense Initiative (SDI) had begun in January of 1984. The importance of the first-ever hit-to-kill intercept was recognized in 1986 when the HOE Program received that year’s American Defense Technical Achievement Award.
The HOE concept never saw mass production since it was a heavy and rather expensive solution to the hit-to-kill problem. However, its technical legacy extends to the present day. Indeed, the highly capable and ever-evolving Aegis Ballistic Missile Defense (ABMD) system is a vital component of our nation’s Ballistic Missile Defense System (BMDS).
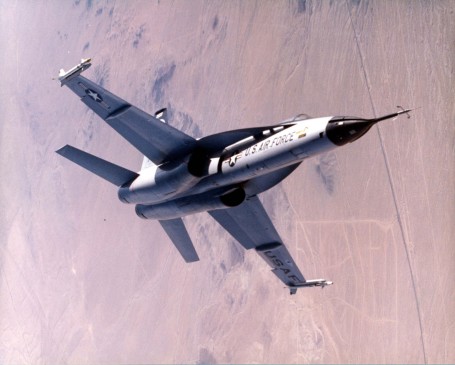
Thirty-eight years ago this month, the No. 1 USAF/Northrop YF-17 Cobra Lightweight Fighter (LWF) prototype made its maiden flight from Edwards Air Force Base, California. Northrop Chief Test Pilot Henry E. “Hank” Chouteau was at the controls of the agile twin-engine jet.
The Lightweight Fighter (LWF) Technology Program was a United States Air Force (USAF) effort to develop a reduced-cost, highly maneuverable combat aircraft. The LWF Program, which began in 1971, ultimately resulted in a competitive fly-off between the Northrup YF-17 and General Dynamics YF-16 in 1974.
The Northrop YF-17 Cobra measured 56 ft in length and had a wingspan of 35 ft. Gross Take-Off Weight (GTOW) was 34,280 lbs. Power was provided by twin General Electric YJ101-100 afterburning turbofans, each generating 14,400 lbs of thrust. The aircraft had a maximum design speed of Mach 1.95, an unrefueled range of 2,600 nm and a service ceiling of 50,000 ft.
The accent on agility and maneuverability led designers to configure the YF-17 with leading edge strakes and twin vertical tails. The leading edge strakes helped alleviate asymmetric vortex shedding and the associated induced yawing moment at high angle-of-attack. Similarly, the twin vertical tails provided enhanced directional stability at high angle-of-attack flight conditions.
YF-17 Ship No. 1 (S/N 72-1569) first took to the air on Sunday, 09 June 1974. The aircraft displayed impressive performance, agility and handling qualitities. On Tuesday, 11 June 1974, the Cobra exceeded the speed of sound in level flight. This marked the first time in United States aviation history that an aircraft did so without using afterburner.
On Wednesday, 21 August 1974, YF-17 Ship No. 2 (72-1570) joined the Northrop LWF flight test force. Together, these two airframes flew 288 flight test sorties for a total of 345 flight hours. During the test program, the Cobra hit Mach 1.95, pulled 9.4 g’s and achieved a maximum altitude beyond 50,000 ft. The jet handled like a dream and lived-up to its advance billing in virtually every way.
Despite the YF-17’s great promise, it did not win the LWF fly-off with the YF-16. Competitions of this sort are often between equals and the final decision can go either way. Nuanced political factors and the like often determine the final outcome. Such was the case in this situation. Both aircraft were exceptional, but only one could be declared the winner.
Happily for American aviation, the YF-17 story did not end with the LWF loss. Indeed, the YF-17’s merits were so obvious to the aviation community that it received new life with the United States Navy. In May of 1975, the team of Northrop and McDonnell Douglas secured the Navy Air Combat Fighter (NAVF) contract to produce a remarkable aircraft that was a direct descendant of the YF-17 Cobra. We know that aircraft today as the F/A-18 Hornet.
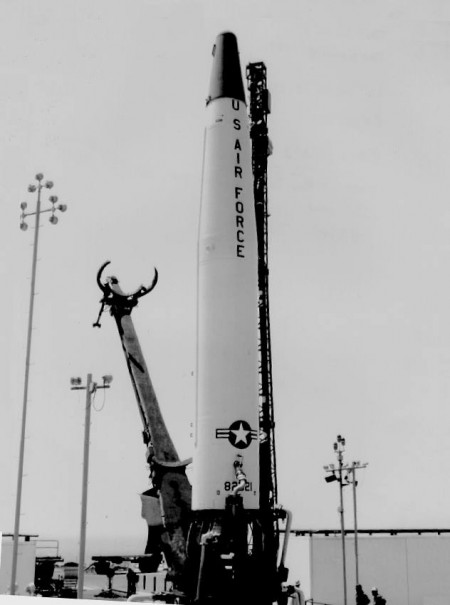
Forty-one years ago this week, the last of the USAF/Boeing Thor Burner II launch vehicle series successfully orbited the SESP-1 space environment satellite. Launch took place from SLC-10W at Vandenberg Air Force Base, California on Tuesday, 08 June 1971.
The Thor Burner family of launch vehicles was designed to orbit classified meteorological satellites for the Defense Meteorological Satellite Program (DMSP). Launched into polar orbit, these satellites aided Keyhole spy satellite operations by ensuring that target imaging took place only over sparsely-clouded terrain.
The liquid-fueled Thor SM-75 missile served as the first stage of the Thor Burner launch vehicle. The Thor airframes selected for the program formerly stood sentinel in Europe in the Intermediate Range Ballistic Missile (IRBM) role. The Burner upper stages utilized solid propellant propulsion.
There were four (4) versions of Thor Burner as distinguished principally by the type of solid propellant rocket motor employed in the upper stage(s) and the orbitable payload mass. Thor Burner 1 used a single Altair-type solid motor upper stage which produced about 5,000 lbs of thrust for 27 seconds. The upper stage and satellite payload were spin stabilized. A total of six (6) Thor Burner I vehicles were flown.
Thor Burner II employed a single Star 37B solid motor which generated 10,000 lbs of thrust for 42 seconds. The upper stage had its own 3-axis flight control system that incorporated multiple hot and cold gas reaction jets. The solid rocket motor and satellite payload were contained within a hemispherically-blunted conical fairing that was colored a distinct orange-red. Thor Burner II flew twelve (12) times.
Thor Burner IIa was a three-stage configuration. A Star 37D (slightly less energetic than the Star 37B) solid motor powered the third stage which was accomodated through the addition of a cylindrical section inserted between the Thor first stage and the hemispherically-blunted conical fairing. Eight (8) Thor Burner IIa vehicles were launched.
The fourth and final Thor Burner variant was essentially a modified Thor Burner IIa vehicle. The second and third stages were now powered by a Star 37XE and Star 37S-IISS rocket motor, respectively. The shape of the external fairing was the same as that of the Thor Burner IIa, only a higher degree of nose blunting was used. A total of five (5) of these vehicles were flown.
The Thor Burner series flew from 1965 through 1980. Twenty-eight (28) of the thirty-one (31) missions were rated as successful. Indeed, the Burner concept proved so effective that variants thereof showed-up on the Atlas and Titan missile programs. Little-remembered today, the Thor Burner Program holds an important place in the annals of American aerospace history.